Quantum physics: The twisted material splits the electrons
In fact, electrons have the smallest possible electrical charge. Four research groups have now discovered particles that contain a fraction of the charge of an electron, without any magnetic field.
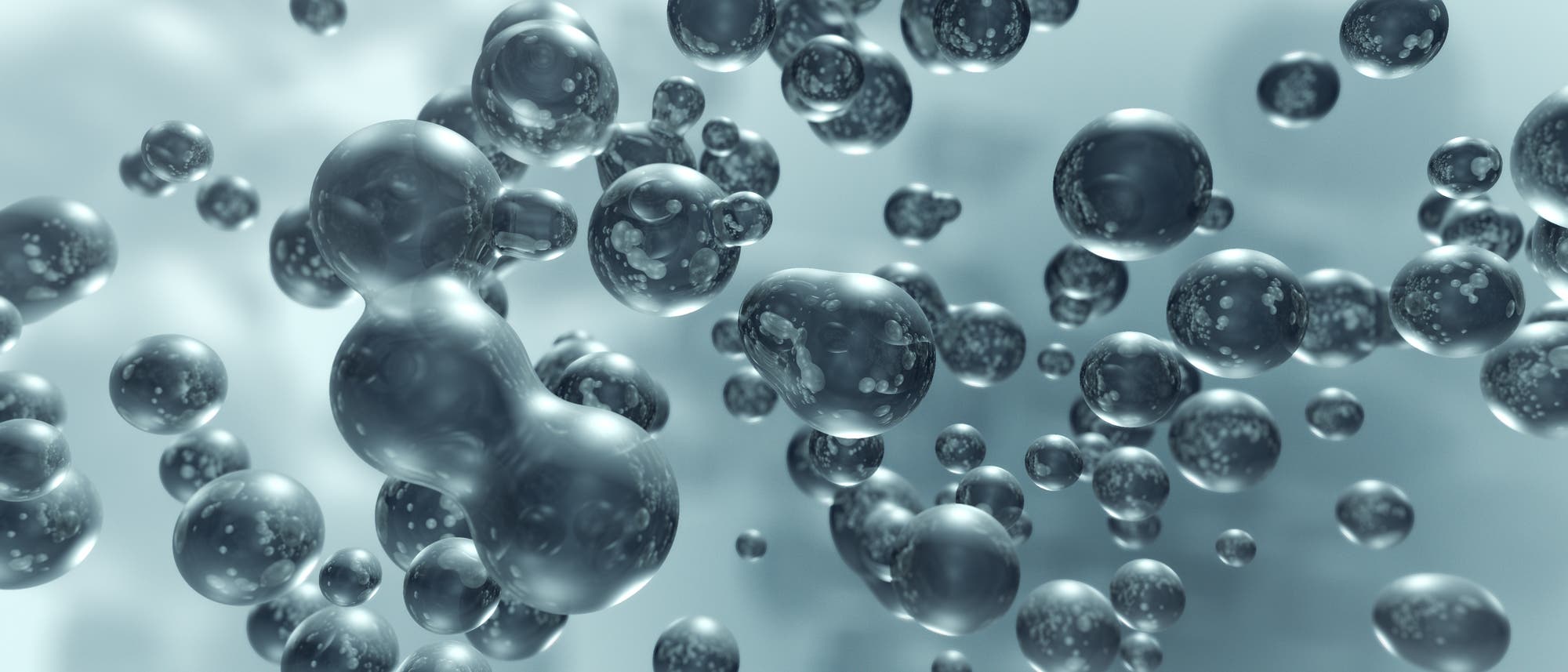
© Friedmantle/Getty Images/iStock (details)
Electrons have no expansion and therefore cannot be split. However, quasiparticles can appear in solids that contain only a small fraction of the electron charge.
The search for new particles is usually associated with giant underground particle accelerators. But it can also be done in regular laboratories where solids are cooled to very low temperatures. Complex interactions between the countless electrons in these materials lead to amazing phenomena.
An example is the partial quantum Hall effect. A strong magnetic field causes electrons in some 2D materials to behave as if they were splitting into three (or more) new particles. These special particles, called anyons, could play an important role in quantum computing in the future. They could enable a new type of quantum computer that is much more powerful than classical models. However, the magnetic field required for this is an obstacle, but there is hope: at the end of September 2023, several research groups succeeded in observing the partial quantum Hall effect without an external magnetic field, as they did in four Different Publications To report.
In most solids, electrons move so quickly that their mutual repulsion is not affected by negative charge. In order to observe the partial quantum Hall effect, the electrons must be slowed down so much that their behavior is dominated by repulsion. This is possible in so-called moiré materials. These consist of several layers of atomic lattices stacked on top of each other, which are slightly twisted against each other. The layers create a pattern of alternating light and dark spots, which represent atoms. Such materials can be made, for example, from 2D graphene layers or molybdenum ditelluride (MoTe2), which is a transition metal dichalcogenide.
Three conditions are necessary for this strange phenomenon
However, to observe the partial quantum Hall effect, the electrons must not only be slow, but also… But it also has topological properties. In this context, the abstract mathematical field of topology has a concrete physical effect: when electrons flow through the material, electrical resistance arises not only along the current (as is usually the case), but also perpendicular to it. In some materials, the Hall effect can occur without an external magnetic field. Experts refer to this phenomenon as the partial quantum anomalous Hall effect (FQAHE) – and its corresponding materials as the partial Chern dielectric.
However, FQAHE requires a third condition that is more precise than the first two: the topological properties must be uniformly distributed among the electrons that make up the electric current.
Finding a material that has the three properties needed for FQAHE has been a major challenge. When moiré materials were first discovered, experts focused primarily on twisted graphene layers. To monitor FQAHE, However, a small magnetic field was always necessary. Twisted graphene is clearly missing the third component of FQAHE: the structure does not appear to be uniformly distributed enough among the electrons. To overcome this problem, the authors of the four studies moved away from graphene and instead turned to a large family of transition metal dichalcogenides.
A different substance as a source of hope
About electrons in MoTe2 To slow it down, you place two layers of 2D material on top of each other and twist them three to four degrees. This creates a stream of anions at the edge of the sample, each of which has a third of the electron charge. High quality mote2Producing samples with such a small twist angle requires a lot of experimental effort.
After the authors of the four studies achieved this feat, they demonstrated FQAH in different ways. Teams led by physicist Jiaqi Cai of the University of Washington and Lihang Zeng of Cornell University examined the electronic properties of the material using light. The electromagnetic field of light affected electronic features in ways that could only be explained by FQAHE. Zeng and his colleagues supported this conclusion by also examining the material’s local electronic compression. However, the group led by Heonjoon Park of the University of Washington and Fan Xu of Shanghai Jiao Tong University took a different approach: they made electrical contacts to directly measure transverse electrical conductivity, a clear indicator of FQAHE.
Solid-state physicists have been eagerly awaiting such an observation. The findings from the four specialized groups could lead to important technological applications. However, there is still a long way to go until the next breakthrough: making anyons available for quantum computation. By controlling the anions, the quantum state of the solid can be controlled, which can be used to store and process information. Such quantum calculations with anyons could be much more powerful than those performed with previous qubits. However, the currently observed FQAHE contain only the simplest types of anions, which are called abelian anions. However, quantum calculations require non-abelian anions, which are much more difficult to understand than those examined in these four studies.
One way to create non-abelian anions is to combine a material with abelian anions using a superconductor. According to theoretical considerations, a superconductor can transform edge currents into non-Abelian instances. Since magnetic fields affect the conductivity of a material, this was not yet possible. Discovery of abelian anions in twisted MoTe2 Without an external magnetic field it can be pioneered. Hybrid components made from MoTe2Layers and superconductors will undoubtedly present new experimental hurdles, but the creative and diligent solid-state physics community must rise to the challenge.
Please allow Javascript to maintain the full functionality of Spektrum.de.
More Stories
Exploding Fireball: Find the meteorite fragments
Neuralink's competitor lets blind people see again with an implant
A huge meteorite has hit Earth – four times the size of Mount Everest